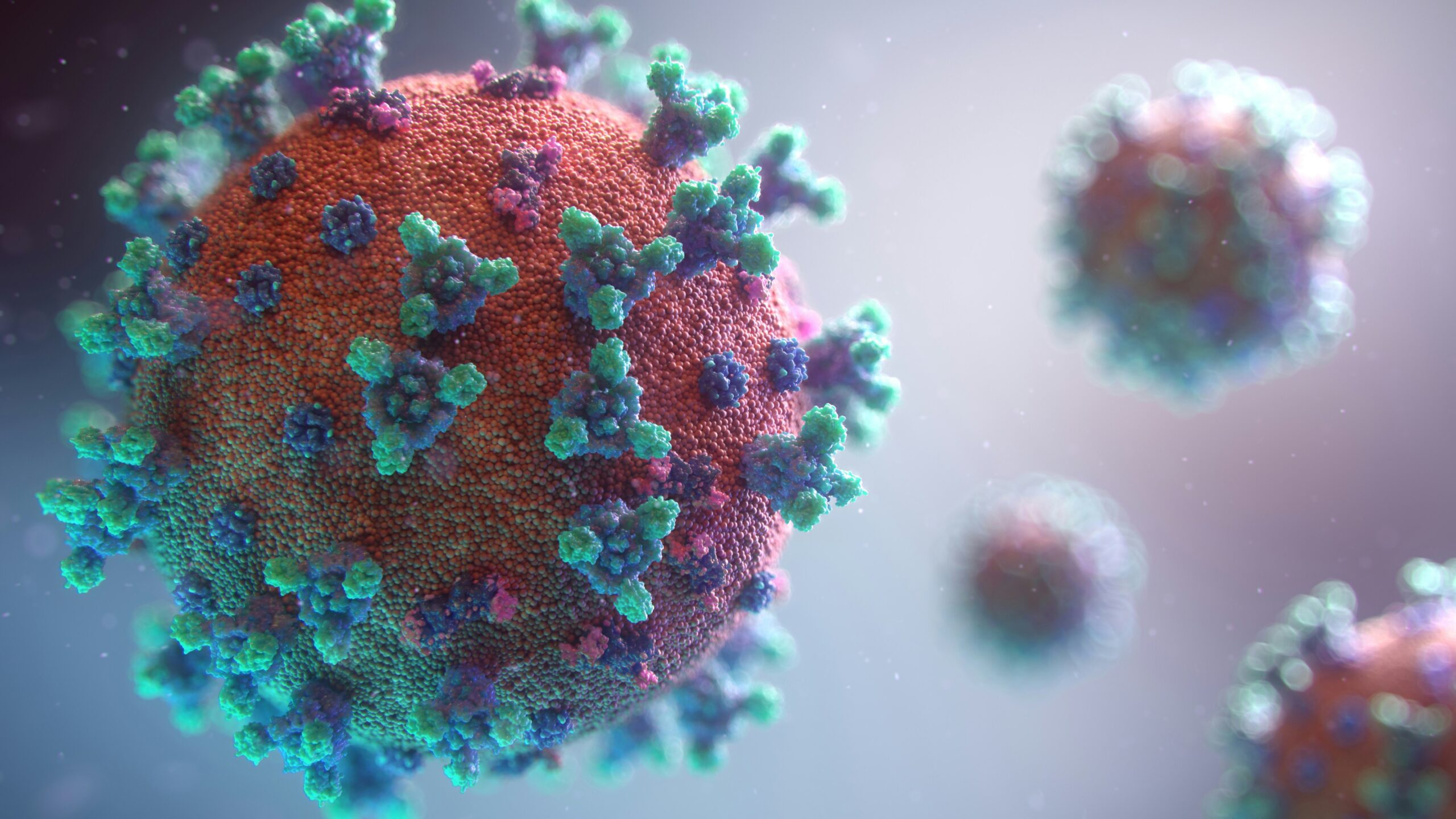
The virus that eats bacteria
Although it may seem counterintuitive, the discovery of phages as a weapon against pathogenic bacteria predates the discovery of penicillin. French microbiologist Felix d'Herelle discovered an "invisible antagonist" that was capable of annihilating bacteria. At first he called it so because it was an organism so small that it passed through the filters developed by Chamberland and Pasteur to remove bacteria from their water samples. However, d'Herelle soon realised that he was dealing with a new type of virus, which he christened "bacteriophage", literally the "bacteria-eating" virus. Later, d'Herelle conducted several experiments which, among other things, showed that phages could infect and kill the bacteria that cause cholera, dysentery and plague. D'Herelle used phages to successfully treat bacterial infections in humans, a revolutionary breakthrough at the time. However, despite this initial success, Western scientists turned their attention away from phages, amazed by the applications and versatility of penicillin as an antibiotic.
Phage research continued in the former Soviet Union thanks to George Eliava, a colleague and collaborator of d'Herelle's who founded a research institute in present-day Georgia. Although Eliava was executed in 1937 by Joseph Stalin's totalitarian regime, Soviet support for research allowed the development of therapeutic phages to continue. It was part of the standard system of medical care in the Soviet Union. Depending on the type of infection, doctors decided whether to use phages, antibiotics or a combination of both, says Russian doctor Mzia Kutateladze in an interview with the BBC. For a long time, this therapy was considered practically pseudoscience in the West, partly because of the political implications of the Cold War, but also because of the lack of access to publications on the subject, which were often hard to find and only available in Russian. After many years in the shadows, phages became famous again in the late 1990s and early 2000s, when scientists at the Georgian institute founded by Eliava succeeded in curing a Canadian patient of an infection caused by a resistant bacterium. These positive results, combined with growing concern about the rise of infections caused by antibiotic-resistant bacteria, renewed interest in phages.
Since then, there has been a significant resurgence in the research and development of phage-based therapeutics. In recent years, significant advances have been made in understanding the biology of phages and in developing techniques to use them as antimicrobial agents. Thousands of different types of phages are now known, specific for killing different types of bacteria and other microbes. This diversity provides an example of the broad spectrum of therapeutic possibilities to combat a variety of bacterial infections.
Antibiotics such as penicillin - and all drugs developed later - are chemical substances, more or less complex, that interact with some of the biochemical processes of bacteria, disrupting their viability. However, phages are viruses, much more advanced structures, capable of parasitising and annihilating bacteria. As biological entities, they have complex, co-evolved relationships with bacteria. Phages have two different types of life cycles: the lytic cycle and the lysogenic cycle. In the lytic cycle, the phage attaches to the bacterial membrane and injects its genetic material into the bacterium. The virus will take advantage of the bacterium's cellular machinery, including its proteins and enzymes, to generate copies of itself and then assemble them, forming new phages - typically between 50 and 200. Once the army of offspring is ready, the bacterium is ruptured - or lysed, hence the name of the cycle - and the phages are released into the environment, ready to spread the infection and attach to new bacteria to start the cycle all over again. In the lysogenic cycle, the phages insert their genetic material into the bacteria's DNA, giving the bacteria immunity against successive infections. When the bacterium reproduces, it will make a copy of its DNA and, with it, copies of the phage's DNA, which remains dormant until conditions are right for it to become 'activated' and begin a process of infection.
In the context of therapeutic uses, phages that can perform lytic cycles are often the most relevant. In this regard, it is therefore necessary to isolate and characterise phages that can be specific and selective against the bacteria responsible for dangerous infections, so that they can be amplified and administered in a way that is safe, but allows them to come into direct contact with the pathogen. For example, in an osteoarticular infection such as the Canadian patient treated in Georgia, it is recommended that the wound be washed with a phage solution before stitching and closed at the end of surgery. The phages, when they multiply in contact with bacteria, will disperse and end the infection as long as there are uninfected bacteria left. Once the infectious bacteria are completely eliminated, the phages, which cannot survive without a host to reproduce, will die as well.
In recent years, very promising results have been presented using phages as therapy in several cases considered critical, which unfortunately is becoming more and more common with the gradual increase of antibiotic resistance. Some studies show that phages can reduce the build-up of resistance in bacteria and can successfully kill infections caused by Staphylococcus aureusone of the most dangerous pathogens if it generates resistance to antibiotics. In addition, the high specificity of phages - able to recognise not only specific species of bacteria, but sometimes even different strains of the same species - allows selective targeting of the pathogen responsible for the infection and preserves the "good" bacteria, technically known as commensal bacteria, that make up our natural microbiota.
Phage therapy has great potential as an alternative treatment to antibiotics, not least because it has a number of very significant advantages over traditional treatments. Unlike broad-spectrum antibiotics, which indiscriminately kill both harmful and beneficial bacteria, phages are extremely specific, and are 'programmed' to target only a specific type of pathogen. This specificity reduces the risk of disrupting the body's natural microbiota, essential for maintaining both physical and mental health. Sometimes this natural specificity needs to be further enhanced in the laboratory, using gene-editing techniques such as CRISPR.
Another advantage of phages is their ability to evolve - co-evolve, in fact - along with bacteria. Bacteria can develop resistance to antibiotics through genetic mutations, and it is precisely this genetic defence mechanism that renders our drugs ineffective over time. However, phages can evolve even faster to overcome bacterial resistance - they are a dynamic and adaptive weapon against resistant pathogens. In addition, phages can penetrate hard-to-reach areas, such as biofilms - communities of bacteria living in a sticky polysaccharide matrix - and infections within the cells themselves, where antibiotics may have difficulty penetrating. This makes phage therapy especially promising for treating both chronic infections and persistent infections that do not respond to conventional antibiotic treatments.
Phages can be administered orally, topically or intravenously, depending on the type of infection and its location. They can also be used in combination with antibiotics to increase their efficacy and reduce the risk of resistance development. In addition, phages have been shown to have immunomodulatory effects, helping to boost the body's natural immune response to infection. Antibiotic-resistant pathogens also work, as demonstrated by numerous studies and clinical trials, including multi-resistant organisms such as Staphylococcus aureus, Escherichia coli y Pseudomonas aeruginosa.
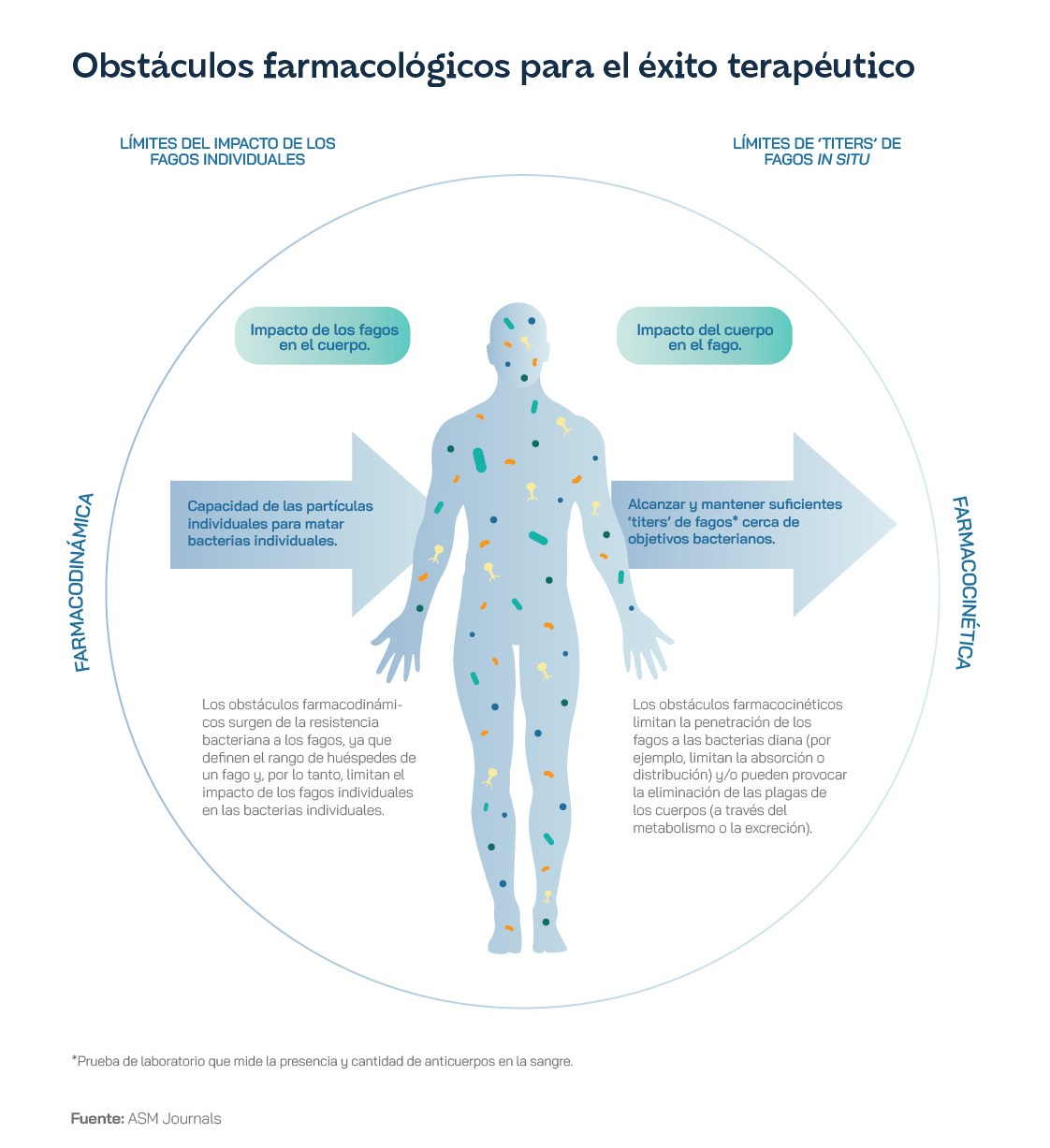
Recent advances in bioengineering - including the advent of CRISPR - have opened up new possibilities for improving the therapeutic potential of phages. Scientists can modify and manipulate the genetic material of phages "a la carte", creating new viruses that are even more selective, more specific but, above all, more effective. It is also possible to programme phages to generate products with therapeutic potential. in situdirectly at the site where they are needed. In this sense, bioengineering phages capable of producing therapeutic proteins improves the efficacy of therapy by modulating the immune response. In addition, new phages can be designed to improve pharmacokinetics - the duration of effects and potency of a treatment - increasing its stability and prolonging its lifespan in the body. Beyond genetic engineering, different types of phages can also be combined into so-called 'phage cocktails', mixtures of multiple phages that attack different fronts of bacterial infection. These cocktails not only improve treatment efficacy by being able to target multiple bacterial strains at the same time, but also reduce the risk of resistance development by preventing the spread of bacteria.
Recent research suggests that phages may have utility far beyond the treatment of infectious diseases, with potential applications in numerous treatments. For example, thanks to the ability of phages to modulate immune system responses, they could be used to treat autoimmune diseases - many of which are growing in prevalence due to numerous lifestyle changes. Several studies are betting on phages to selectively target immune cells involved in autoimmune disorders such as rheumatoid arthritis or inflammatory bowel disease. By eliminating these cells, phages could help dampen the immune response - and, perhaps more importantly, the inflammatory response - characteristic of these conditions. Phages could also become a powerful tool in the fight against cancer. Through genetic engineering, the behaviour of bacteriophage viruses could be modified to carry and inject therapeutic payloads directly into cancer cells. This could include the delivery of chemotherapy - usually cytotoxic substances that kill cancer cells - and immunotherapy - the use of proteins and antibodies that act as immunomodulators and stimulate our immune system's natural response against tumours.
Phages could also be used in applications such as cancer gene therapy, which typically uses more complex viruses to carry RNA and DNA fragments with therapeutic potential. This can be achieved by modifying the phage's surface proteins so that they are able to specifically and selectively recognise and bind to certain cancer cell markers and receptors. Now that we also have a better understanding of the interactions between the gut microbiota and the development of certain types of cancer, phages could be designed to target specific bacterial populations within the gut if they are associated with tumour growth or metastasis.
Despite the promising results of recent phage studies, this therapy still faces disbelief from many on the one hand, and lack of funding and medical regulation on the other. A few years ago, a clinical trial led by French researchers studied phage therapy in infected burns, funded by the European Commission with 3.8 million euros. However, this study had to be stopped due to a number of problems, including difficulty in recruiting patients and poor stability of the prepared phages. In addition, preliminary results indicated that the phages did indeed help reduce bacterial infection in some patients, but much more slowly compared to standard antibiotic treatment. This setback may have slowed the uptake of phage therapy in France and, consequently, in the rest of Europe.
But there is still reason for optimism: Belgium has taken a significant step by approving the use of phages as personalised medicines, prepared by a qualified pharmacist if there is a medical prescription. Jean-Paul Pirnay, a Belgian researcher, believes it is "only a matter of time" before personalised phage therapy becomes a standard treatment option worldwide. Pirnay and other scientists in the field suggest the creation of a new regulatory system that would allow the development of a catalogue of tested and certified phages, as well as 'seeds' and dormant phages, both of which are necessary for the creation of personalised preparations. It is clear that, despite their great potential, phage applications are still at an early stage, where there is still a long way to go in terms of research and development, regulation and legislation. Investment in these therapies is essential, especially considering the global antibiotic resistance crisis we are facing, in order to fully understand the mechanisms of action of phages and to develop effective and safe mechanisms for their application and commercialisation.
The difficult transition to phage medicine
The OECD estimates that EU and European Economic Area countries will spend up to €1.1 billion a year on antimicrobial resistance healthcare if the rising incidence goes unchecked, including costs arising from slower recovery from infection and those associated with increased risk of complications, with up to an additional €569 million for hospital days. The World Bank estimates that antimicrobial resistance could cost the global economy between one and 3.4 trillion dollars a year by 2030. The use of phages to combat these pathogens is presented as one of the most attractive alternatives to conventional therapies, but it still has several weaknesses. For a start, it can be difficult and time-consuming to identify which pathogen to eradicate, which phage is needed, and its dose, potency and capacity. The interrelationship between a phage, the bacteria and its modification after contact with each other and the environment can also be particularly complex and difficult to determine. In addition, the costs of formulating and stabilising pharmaceutical-grade phage preparations differ depending on which variants are used and, as with antibiotics, there is the potential for bacteria to develop resistance to phages. Ultimately, because phages are not self-antigens but originate outside the host body, they may be recognised by a patient's immune system and attacked, reducing their efficacy, if not directly leading to anaphylaxis, an autoimmune response in patients.
Despite all this, in some countries, notably Russia and its exsatelies during the Soviet era, phage preparations have become registered medicines, with dedicated research centres. In Georgia, patients are treated with generally manufactured phages or with individualised phages, if the bacteria are resistant to standard variants. Phage products are also used for environmental protection, sanitation and decontamination of different environments contaminated with bacterial pathogens. The Georgian biopharmaceutical company BioChimPharm completed the modernisation of its historic bacteriophage factory in 2022 with financial support from the EU, the EBRD, the FAO and its Ministry of Economy and Sustainable Development. In Poland, the Phage Therapy Unit (PTU) at the Hirszfeld Institute of Immunology and Experimental Therapy in Wrocław has been treating patients since the 1950s and has been allowed to continue using phages on an experimental treatment basis after joining the EU in May 2004. Since then, and until 2020, more than 700 patients were treated at the OCT. In fact, it has been producing them for patients throughout Europe and North America. And the universities of Jyväskylä and Helsinki have long been researching phages and, in some cases, have provided them for emergency treatment. Through its investment tool Unfund has leveraged PrecisionPhage to support its international expansion.
Other EU countries have shown clear signs of interest in this biomedical field in recent years, but the reality is that, as of early 2024, there were no phage-based medicines approved under EU law for human use. In Slovakia and the Czech Republic there was one, Stafal, but it entered these countries before both joined the EU. When phage therapies are used in Member States, it is generally at the level of an individual experiment or as part of a clinical trial. The only exception would be the magistral approach approved in Belgium to facilitate phage therapy. It consists of a three-page document that "describes how to produce a phage and perform quality control", and is signed by a physician.
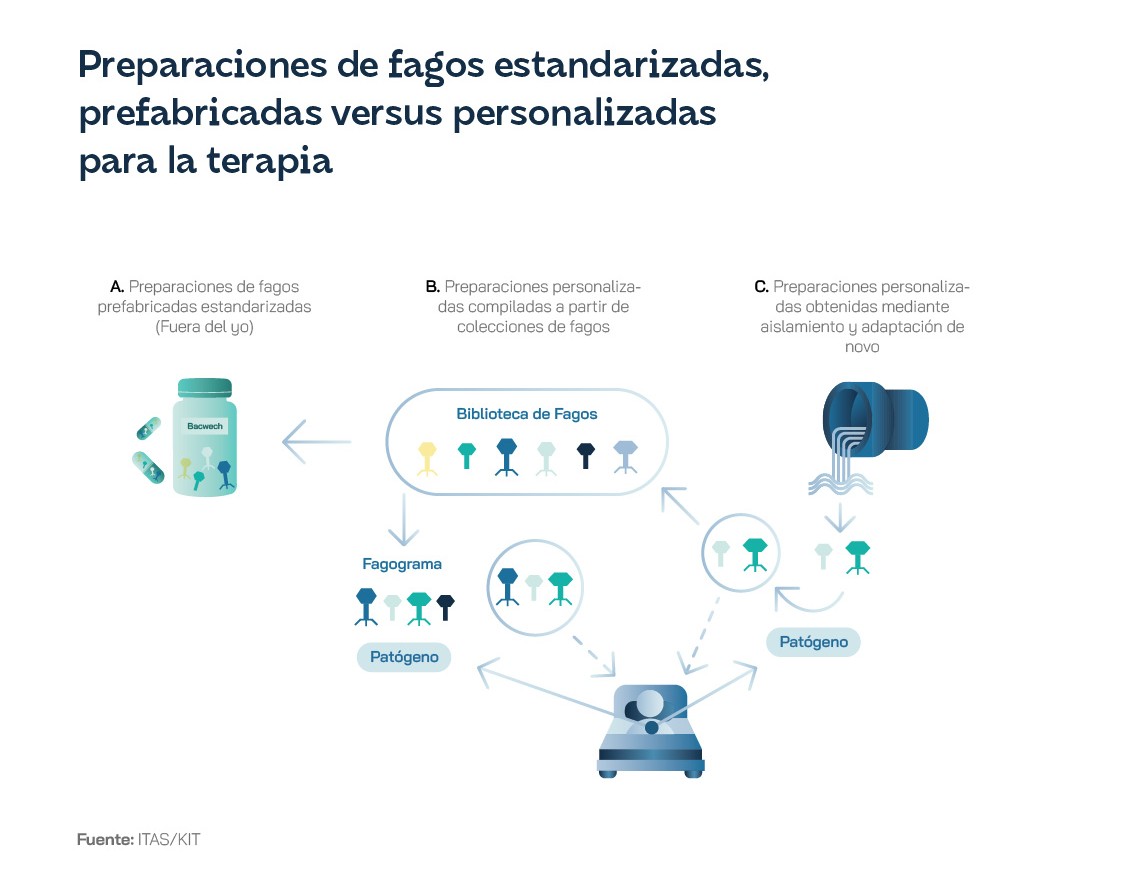
Poland and Belgium have consequently taken the lead in the EU. Voted Europe's most innovative life sciences startup in 2021, Belgium's Vésale Bioscience received €1.8 million from the European Innovation Council to develop its pioneering therapy platform that produces personalised phages for individual bacterial infections in a rapid turnaround time of less than 24 hours, meeting the requirements of the Good Manufacturing Practice (GMP) standard. Meanwhile, France and Germany have opted for the longer route and are trying to promote standard, not personalised, but general-purpose treatments for bacterial infections.
The winter of the phages The United States and Western Europe appear to be winding down, but asymmetrically. The emergence of antibiotics in the 1920s led to a decline in interest and therapies were limited to a small number of experimental treatment centres with almost individual clinicians and researchers. In the UK, they have been used on only 12 occasions in the last four years, in two patients with cystic fibrosis at Great Ormond Street Hospital and in 10 patients with diabetic foot infection at two Scottish hospitals. In all cases where they have been tested outside the orbit of Eastern European countries, phages have been permitted as medicinal products without a licence, or under specific national rules that have allowed them to be prescribed by medical professionals, but not manufactured for wider use, as is the case in Belgium.
Phage therapy has proven successful in treating life-threatening infections, with results ranging from complete elimination of infection to substantial improvement in patient outcomes for conditions ranging from cystic fibrosis and pneumonia to prosthetic, bone, joint and urinary tract infections. This is why the US government's National Action Plan to combat antibiotic-resistant bacteria, 2020-2025, had to recognise their curative potential and included phages in its call to accelerate basic and applied research and develop new therapies.
The European Parliament recognised the great potential of phages in its resolution on EU action to combat antimicrobial resistance, but limited itself to calling for an appropriate regulatory framework for the registration of bacteriophages as feed additives and veterinary medicinal products. In 2023, the European Medicines Agency took an important step in this direction by publishing a framework of conditions for the authorisation of phages as animal medicines. This decision was used by the organisation PhageEU to call for the development of similar frameworks so that a wide range of phage products could enter the European market. In the European Commission's proposal for a new directive on medicinal products for human use, presented in April 2023, phages were mentioned in this category. As for the possibility of transgenic phages, the EU has strict regulations on genetically modified organisms, which could offer other countries a competitive advantage in developing and exploiting this line of treatments.
In the regulatory field, there is still a long way to go. In France, phages are obtained in a similar magistral-type preparation, which is provided by Pherecydes Pharma or the Queen Astrid military hospital in Brussels to a hospital pharmacist. The National Agency for the Safety of Medicines and Medical Devices must validate the use of these phages through a committee of experts. According to the doctrine of the CJEU, phage therapy can be assumed to have a pharmacological effect within the meaning of pharmaceutical law and, at the level of EU law, there is no ban on phage therapy. Each Member State is competent to give the green light for treatment. However, harmonisation of phage medicines would require a mandatory centralised EU authorisation and a provision similar to the hospital exemption to ensure that, for individualised phage medicines, national traceability and pharmacovigilance requirements and specific quality standards are equivalent to those applied at EU level. In addition, it should be technically and legally unequivocally stated that they are biological medicinal products.
Doubts about its deployment in healthcare systems are reflected in estimates of the potential economic scope of the global phage therapy market, which range from $167.71 million in 2031, according to the most conservative forecasts, to $1,651.8 million in the more expansive ones that include applications in food and beverages. Investors are asking why they should put money into a technology that has been around for 100 years but has not been widely adopted outside the former Soviet Union. What makes the current moment different from others in the past is genomic analysis, which is much easier and cheaper than in the Soviet era, allowing researchers to better select and design the right phages.
It can be the key to creating the institutional environment that this technology needs to scale. In November 2022, Innovate UK established a Phage Knowledge Transfer Network. The Medicines and Healthcare products Regulatory Agency planned to publish draft guidance by the end of 2024 on the licensing of phage products, which could finally clear up doubts about their regulation in the UK. The UK health agency's National Type Culture Collection is the oldest bacterial strain library in the world, and its new repository is intended to be a trusted source for scientists to access and deposit phages.
All will depend on clear evidence of clinical efficacy. As of May 2024, there were 78 phage trials open worldwide, according to Clinicaltrials.gov. In the 59 conducted between 2000 and 2021, 79% of patients with chronic, drug-resistant infections showed improvement, while 87% of target bacteria were eradicated. Often cited is the EU-funded Phagoburn experiment, which worked on a phage product containing anti-Escherichia coli and anti-Pseudomonas aeruginosa bacteriophages to fight infections in burn patients. Phage Australia launched an innovative clinical trial to evaluate the delivery protocol and monitoring of phage therapy, a step beyond models focused on single phages or cocktails. This approach would allow customisation to individual patients' needs, while standardising treatment methodology and data collection. However, other studies raise doubts about their success as antimicrobials and also report eloquent failures, including a compelling report by the Scottish Health Technologies Group, or the documented improvement and subsequent death of a phage-treated patient. Research conducted over decades in Eastern Europe is numerous, but would not meet today's rigorous standards. To this must be added the low level of awareness among physicians. A deeper understanding of phage biology and host range would be necessary to develop more effective phage-based methods and appropriate diagnostic tools.
A major challenge for the development and use of phages is also the lack of manufacturing capacity, mainly because current regulatory processes seem inadequate for this. In most Western countries they are classified as a pharmaceutical product requiring GMP standard, which implies a complex, costly and time-consuming approval process. The phage's status as a biological entity, which also often appears in cocktails with other phages and other agents, such as antibiotics, and is susceptible to genetic modification, does not help to simplify matters. Phage therapies are often designed to treat specific bacterial infections and even customised for individual patients, in contrast to the fixed pharmaceuticals associated with the GMP model. Hence, with the potential for phages to be widely used, the case is being made for a flexible and customised drug licensing regime, allowing a unique combination of phages, generic and designer phages, often with antibiotics, and providing the necessary regulatory certainty for their production and manufacture. The most appropriate solution is likely to be to promote large-scale GMP production for generic phages and individualised solutions for patients requiring specific combinations. This technology requires an agile response, which is not provided for by current European regulations, whereby the necessary periodic updates of phage cocktails to counter phage resistance would require new, lengthy and costly marketing authorisation applications.
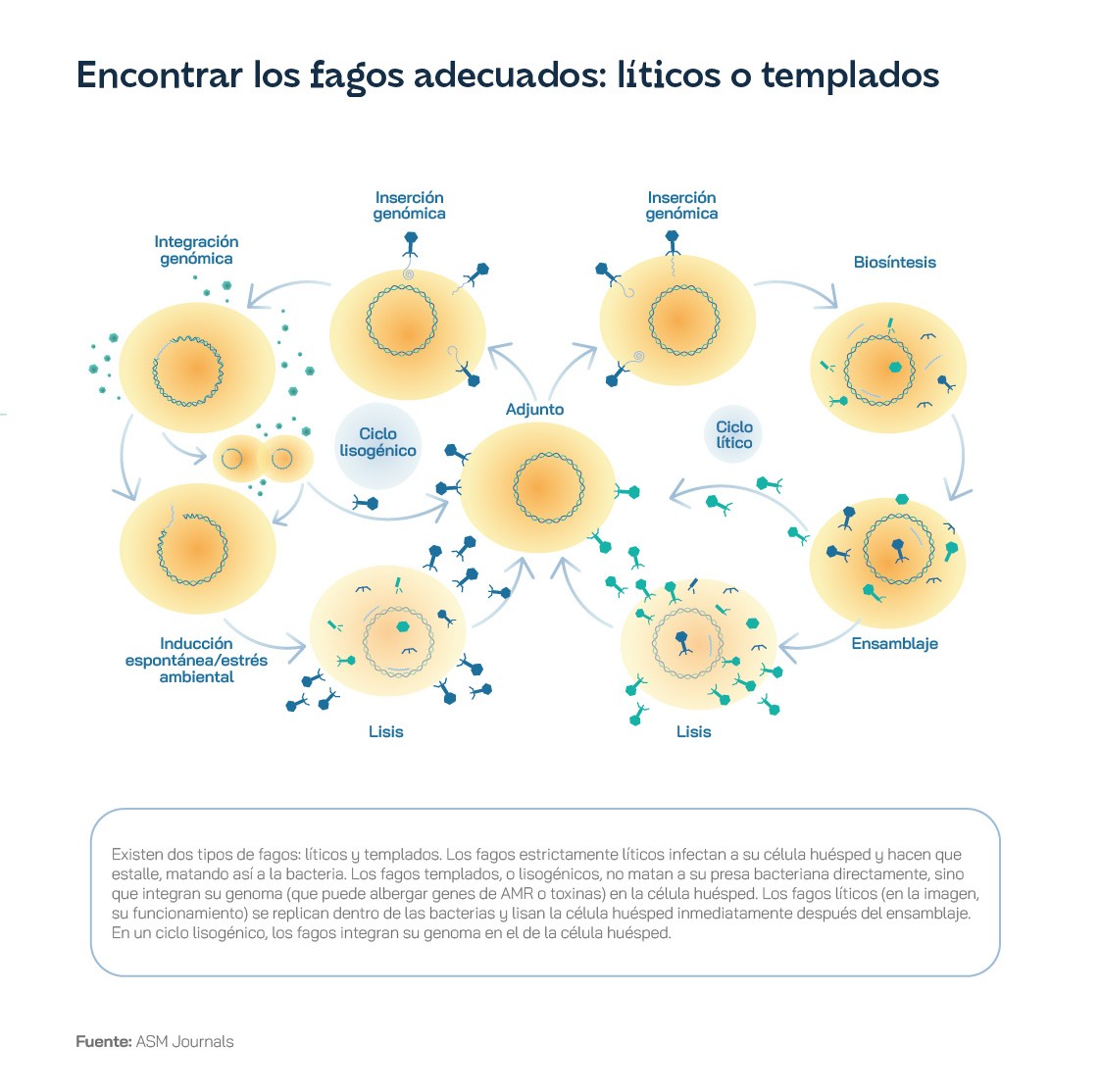
200,000 to produce each individual phage to GMP standard, and at a more advanced stage around Ultimately, the UK estimates that in the event of a GMP facility going live, with an investment of between 23.5 and 35.2 million euros, and taking into account economies of scale, the cost could be reduced to a couple of pounds per patient and savings to the health system of between 175 and 230 million euros per year in the treatment of diabetic foot, hip and knee infections alone could be achieved, through reduced antibiotic use and fewer interventions such as amputations and other surgeries.
At the beginning of 2024, only Slovenia, Portugal, Norway and the United States had GMP phage facilities. The AMR Action Plan 2024-2029, which set out the UK government's research priorities, included innovation of new products to tackle antimicrobial resistance. One such stimulus for industry could be to facilitate the patenting of unique phage combinations and modified phages. Access to robust clinical trial data to help bridge the so-called "translation gap", i.e. to boost access to molecular genetic, sequencing and computational biology tools to ensure that phages developed for therapy are safe and effective, would also help.
The World Health Organisation insists on a One Health approach and tackling antimicrobial resistance from a broad perspective that includes human and animal health, the food chain and the environment. The reality is still far from making such a vision possible at the systemic level. For example, for phages to be transported efficiently between countries, there should be a well-established, regulated and up-to-date register, but this is not the case. National and institutional information is today published sporadically in a variety of scientific journals. A One Health approach would also involve transdisciplinary cooperation, because there is much to learn from research on the application of phages in agriculture. It has been shown, among other useful contributions to the health sector, that the use of phage preparations in pre-slaughter practices decreases pathogenic bacterial contamination of meat for human consumption. In the current context, obtaining high quality phages is difficult, especially for those unfamiliar with the therapy. Access to phages is informal and largely driven by networking between clinicians and phage laboratories. It is via disparate, generally non-commercial, international sources and relies on payment per patient of up to 12,000 euros for phage isolation and characterisation.
Too much risk for investors. Although the World Economic Forum listed the use of bacteriophages as one of the top 10 emerging technologies of 2023, it is often difficult to get funding for clinical trials, especially from pharmaceutical companies, due to their high cost, especially when they move to phase II and phase III. And without clinical trials, countries that fail to obtain data at home will have to use clinical data from other countries or from non-health sectors, such as agri-food. The FDA is releasing funds in the US and the National Institutes of Health allocated a first tranche of funding of $2.5 million to 12 institutes around the world to boost testing. In June 2018, the Innovative Phage Therapeutics and Applications Center (IPATH) opened at the UC San Diego School of Medicine with the aim of providing phage therapy to patients with multidrug-resistant infections under the FDA's compassionate use programme. The startup Armata Pharmaceuticals received about $16.3 million from the US Department of Defense for research on the S. aureus bacteriophage in relation to antibiotic resistance in Ukraine. Scientific and technological work is progressing while the institutional sphere is undergoing its own transition.
Initiatives to reach out to health from the field
The only phage clinical trial involving Spanish research entities in 2023, according to ClinicalTrials, sought to prove the validity of a bacteriophage-based therapy for patients with cystic fibrosis and chronic lung infection by pseudomonas aeruginosa, a type of aerobic bacterium. The Hospital Vall d'Hebron in Barcelona and the Hospital Clínico Universitario Virgen de la Arrixaca in El Palmar (Murcia) were present, and the participants had to be older, clinically stable patients receiving standard cystic fibrosis medication.
In the field of basic science, the Translational and Multidisciplinary Research Group of the Biomedical Research Institute of A Coruña (INIBIC) of the Hospital A Coruña has developed several lines of innovation in phage therapy, and has been awarded by the association of biotechnology companies Asebio for the application of the strategy of bacterial anti-persistence, the main mechanism used by bacteria to defend themselves against infection by phages. His work focuses on the efficacy of natural lytic phages combined with enhancer treatments, and the development of synthetic phages that carry proteins of interest to favour their activity.
In Spain, it is more common to find research on phages in the agri-food field, an aspect that, as we have seen, may offer possibilities for subsequent application to humans. The C-SNIPER project seeks to obtain an innovative, natural and efficient bacteriophage-based solution to reduce the prevalence of the Campylobacter in poultry. It causes campylobacteriosis, the most common foodborne disease in Europe and the most common route of human infection, with an estimated associated cost of €2.4 billion per year.[4]. However, there are no phage-based products on the market to combat it, as is the case for other food-borne pathogens such as Listeria monocytogenes, Escherichia coli and Salmonella. Current control measures, based on the use of antibiotics at farm level, are leading to the spread of strains of Campylobacter multi-resistant bacteria. For this reason, the European entity EIT Food decided to finance the C-SNIPER project, in which the Spanish scientific and technological centre AZTI, the Institute of Animal Reproduction and Food Research PAS (Poland), the University of Turin, the Phage Technology Center and ORA Società Agricola are participating.
Researchers from the Universitat Autònoma de Barcelona also took part in the European PhagoVet project to develop three products based on bacteriophages to fight Salmonella and Escherichia coli as an alternative to the use of antibiotics in animal production. The project proposes the use of bacteriophages to control these pathogens in poultry production.